By Dan Rubinstein
Photos by Justin Tang and CERN
At 46 metres long, 25 metres high and 25 metres wide, the 7,000-tonne ATLAS detector is the largest particle detector ever built. It’s one of two general-purpose detectors and one of four major experiments at the Large Hadron Collider (LHC) particle accelerator, a 27-kilometre ring of superconducting magnets buried beneath the French-Swiss border near Geneva.
ATLAS played a central role in the 2013 Nobel Prize-winning discovery of the Higgs boson particle, a breakthrough that confirms the Standard Model of physics and helps us understand how the basic building blocks of the universe acquire mass.
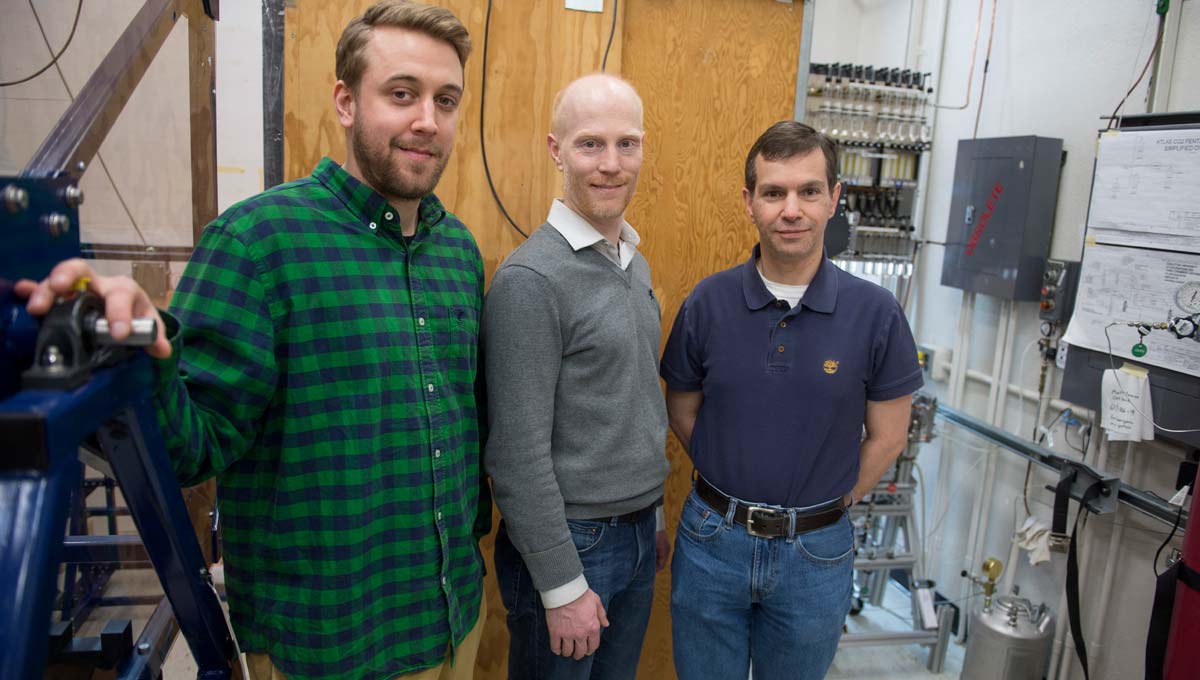
Scientists are using the detector’s precise measurements to improve our understanding of the fundamental constituents of matter, to explore the fundamental forces of nature and to search for new physics phenomena that don’t mesh with the Standard Model.
But the ATLAS inner tracker — the first part of the module to see the decay products of the high-energy collisions between particles, and the largest component of ATLAS — is starting to deteriorate.
CERN, the European Organization for Nuclear Research, knew this would happen when it conceived of ATLAS in the 1980s and turned the detector on for the first time in 2008. The collision area at the heart of ATLAS is one of the most radioactive places on the planet, and that takes a toll on the highly sensitive equipment used to measure “the direction, momentum and charge of electrically-charged particles produced in each proton-proton collision.”
Carleton physicists have been working on the ATLAS experiment since its inception, and now a multi-disciplinary team at the university have a hand in the upgrade project that will replace the inner tracker as the next phase of research at the LHC begins.
“The inner tracker will die a natural death at some point in the next decade,” says Prof. Thomas Koffas, who came to Carleton in 2011 after seven years on the research staff at CERN.
“We knew we would have to replace it sooner or later. Now the goal is to replace it by 2023. It’s the ‘guts’ of the whole detector. Without it, we cannot do the physics.”
A Massive Upgrade for a Massive Project
The scale and complexity of the LHC and ATLAS experiment are truly impressive.
Running the LHC requires more energy per second than it took to sail the 342-metre-long USS Enterprise — a recently decommissioned U.S. Navy aircraft carrier that is the longest warship ever built — at 55 kilometres an hour. CERN is such a major consumer of power, in fact, that the accelerator doesn’t run during the winter; people who live in the area need that electricity to heat their homes.
As the CERN website explains, ATLAS “consists of six different detecting subsystems wrapped concentrically in layers around the collision point to record the trajectory, momentum and energy of particles, allowing them to be individually identified and measured….
“Over a billion particle interactions take place in the ATLAS detector every second, a data rate equivalent to 20 simultaneous telephone conversations held by every person on the earth. Only one in a million collisions are flagged as potentially interesting and recorded for further study.”
The experiment is being conducted by about 3,000 scientists from 180 institutions in 38 countries — “one of the largest collaborative efforts ever attempted in science.”
The $370-million ATLAS-ITk (inner tracker) upgrade also requires a massive partnership. Koffas and colleagues in Carleton’s Physics and Electronics departments are working with researchers at eight universities across Canada and 90 other institutes around the world.
They’re currently in the R&D phase of the project, but based on results acquired over the past decade, the upgrade team knows what changes they need to make.
For starters, says Koffas, all of the active elements in the new tracker will be made from silicon, whereas previously only the inner core was silicon. This will allow researchers to reconstruct the trajectory of charged particles more precisely, a granularity that will help them learn more about key LHC events.
Moreover, because the LHC itself is scheduled for an upgrade in the early 2020s — a process that will give the accelerator more power and create more collisions — the ATLAS inner tracker must be able to cope with the LHC’s enhanced performance and last for at least 10 years.
The ATLAS detector is more complex than the space shuttle, says Koffas. It may not have to fly, but it must be robust enough to be left alone and work for a decade.
“I see ATLAS as a series of smaller experiments,” he says. “You put in effort and there are results, and there is excitement and gratification at every stage. Each step has its own rewards.”
Koffas’s colleague Prof. Dag Gillberg, who joined Carleton’s Physics Department last year after three years as a research fellow at CERN, is focused on the measurement side of ATLAS. Though he’s up to speed on the project’s progress, he’ll get really involved when the new detector is up and running.
“We need all sorts of different skills on our team,” says Gillberg. “The LHC can be most effective if we really ramp up the amount of data that is produced and look for everything in as many different ways as possible.”
“We know there are a lot of things we don’t understand about the universe,” says Koffas, “so we have to keep on looking.”
An Investment in Our Understanding of the Universe
Canada’s and Carleton’s focus for the ATLAS-ITk project revolves around 20,000 silicon strip modules that will have to be built between 2019 and 2023.
These modules consist of sensors, circuit boards and readout chips, and allow the inner tracker to record the positions of charged particles with extremely precise accuracy. Between 1,000 and 2,000 sensors will be tested at Carleton and then distributed across the assembly sites — the university will be the North American entry point for sensors.
In the meantime, Carleton physicists are already collaborating with colleagues in the university’s Department of Electronics on the design and production of silicon strip modules.
Carleton’s MicroFabrication Facility, a cleanroom lab in the Minto Centre for Advanced Studies in Engineering, will be used to probe the sensors and conduct research on their effectiveness.
Modules that allow the inner tracker to record the positions of charged particles will be built in Carleton's MicroFabrication Facility.
The facility, which has been operating since the late 1960s, has decades of accumulated expertise on developing, assembling and testing semi-conductors and other electronic devices. It has a long relationship with medical physics researchers at Carleton, says Department of Electronics Chair Niall Tait, and is only starting to work with particle physicists.
“Most of the detector design has been done, so to a large extent this will be an assembly activity,” says Tait.
“But there are research aspects — these devices have a fair number of parts, have to be 100 per cent reliable and have to be assembled in a reasonable amount of time.”
Moreover, electronics students could be called upon to help refine the signal processing and data collection techniques, and Tait sees the potential for further cross-pollination.
“Anytime you develop a capacity to do different things, new applications are likely to appear,” he says. “I could imagine perhaps looking at applications in security — a similar detector could be used to monitor radiation. A similar technology might be applied.”
“This technology is new for everyone,” adds Koffas, “and it has applications in aerospace, medical physics, nuclear physics and other fields. If the chips can survive at the LHC, they will survive at a nuclear facility.
“It’s so complex, how the electronics are being designed, how they will gather the particle physics data. Because of this complexity, there are applications to big data, Internet banking. When you do a physics experiment of this magnitude, you can branch out in so many directions.
“We’ve never done anything with them before research-wise,” Koffas says about the interdisciplinary partnership with his electronics colleagues. “Once we learn how we can work together, we will see if there are other common areas of interest. This project could be a trailblazer.”
ATLAS Upgrade a Team Effort
Carleton’s ATLAS-ITk team is also collaborating with Canadian companies such as Ottawa-based DA-Integrated, which designs, develops and tests integrated circuits.
Integrated circuits will read the low-level electronic signals from hundreds of sensors at the core of the ATLAS detector, and will manage hundreds of megabits of data on the way toward further processing.
DA-Integrated will assemble and test tens of thousands of circuits for ATLAS over the next few years, and Alex Edwards, the company’s director of business development, is already thinking beyond this project to its broader implications.
“The advanced micro-electronics that are being developed and used for this system, and the advanced manufacturing techniques, are in so many products we use as consumers,” he says, citing the telecommunications and oil and gas industries as just two examples.
“Working on these types of projects can really help Canadian companies broaden skillsets, which we need to compete in international markets.”
Projects such as ATLAS-ITk also provide educational opportunities for students like second-year Carleton master’s student, Robert Hunter, who will be continuing with this work for his PhD.
Hunter spent about a month and a half at CERN last summer, which gave him a sense of what this complex multi-faceted project is working toward. His role, supervised by Koffas, is on the hardware side — helping to test sensors before they go into the silicon strip modules.
“Because it’s such a large project, there are many levels of quality assurance and these preemptive tests are extremely important,” says Hunter.
“Personally, I like the hands-on work — to be able to physically test these devices in the lab, to hook them up to the equipment, develop the software, and see if they’re doing what they’re supposed to be doing. These projects require a carefully measured synergy of widely variant, technical fields and a robust experimental test setup is integral to understanding all of these interactions.”
Carleton students participating in the project are also learning how to collaborate internationally and partner with industry, and how to work with people at other Carleton departments.
“Students will be able to see how a detector is built, which is not something that happens every day,” says Koffas. “When you try to teach someone how to become a blacksmith, it’s one thing to show them a PowerPoint and something else to give them a hammer and an anvil. That’s what we’re doing.”